
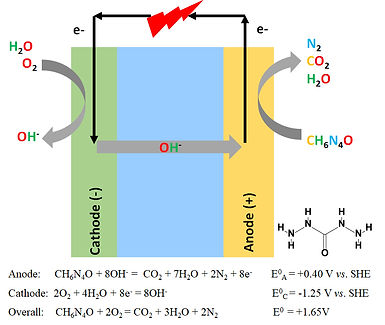
Direct liquid fuel cells are considered an ideal electrochemical energy device to supplement Li-batteries in some special applications, due to the higher energy density of liquid fuel, quiet operation, and independent of charging plugs. We are interested in develop direct carbohydrazide fuel cells with high power density and high energy and fuel efficiency. Now we are investigating electrochemical reaction mechanisms of carbohydrazide, hydrazine and ammonia over different types of catalysts in various conditions, and exploring efficient anode catalyst materials, with the ultimate goal of developing novel carbohydrazide fuel cell technologies.
Sponsor: Iowa Reagents Innovation Fund (RIF)
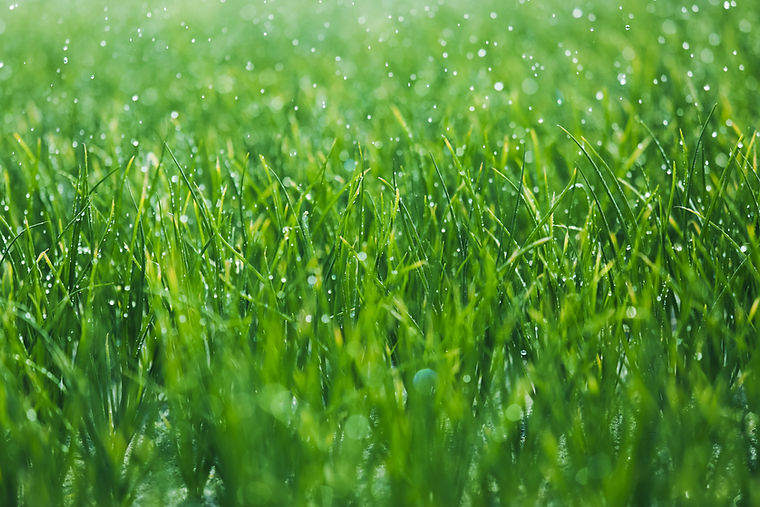
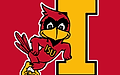
Wenzhen Li Research Group
Electrochemistry / Catalysis / Energy
/ Environment / Agriculture / Sustainability
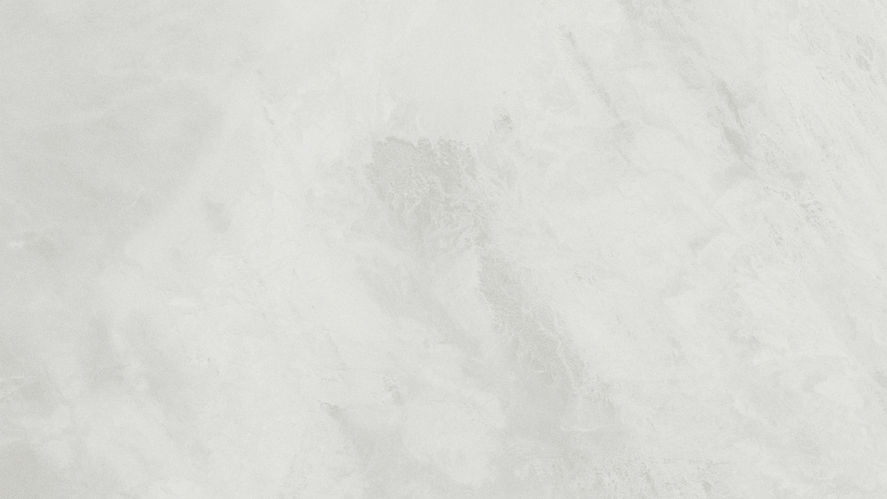
Overview
Electrocatalysis and Electrochemical Systems are Key for a Sustainable Future
Welcome visiting the website of Wenzhen Li research Lab at Iowa State!
Clean energy, sustainability, and advanced manufacturing are our grand needs. Innovations in these areas have the potential to transform our nation’s prosperity and security. Advanced electrochemical systems such as fuel cells, batteries and electrolyzers can generate, store, and utilize renewable electricity, thus enabling distributed, flexible and modular chemicals manufacturing towards a sustainable future.
Our interdisciplinary research is at the interface of electrocatalysis science and engineering. We have strong interest in elucidating electrochemical reaction pathways and mechanisms, and based on the acquired understanding, we rationally design electrocatalysts and electrochemical processes / devices for important energy, environmental and agricultural applications.
Our current research activities include development of advanced electrocatalysts, novel electrochemical processes, electroreduction of nitrate to valuable nitrogen-containing chemicals, carbon dioxide capture and electroreduction, electrosynthesis of nitrogen fertilizers, innovative electrolyzers for hydrogen production, and paired electrolyzer design.

Current Projects
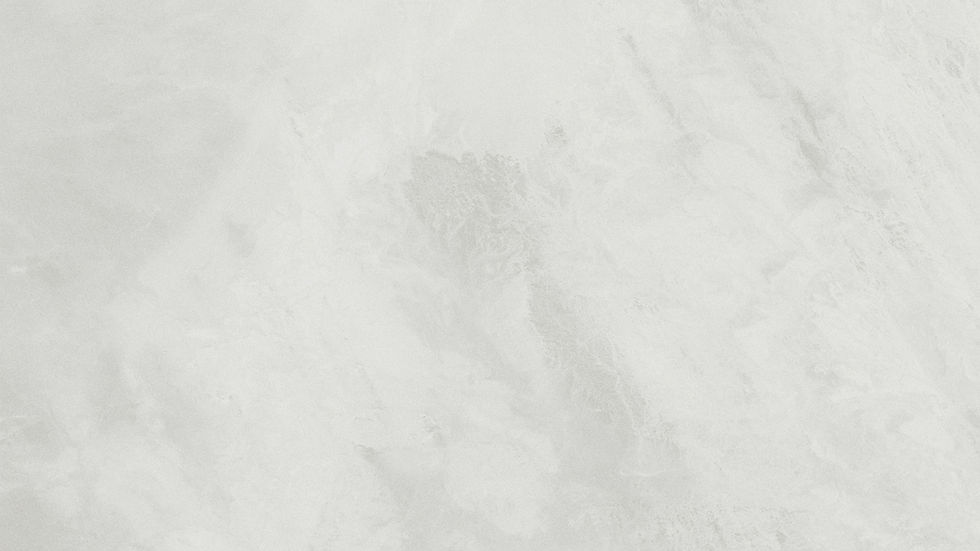
Electrocatalytic Conversion of Biorenewable Compounds
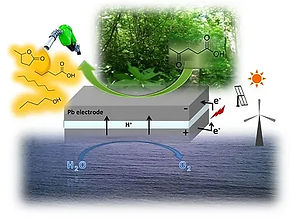
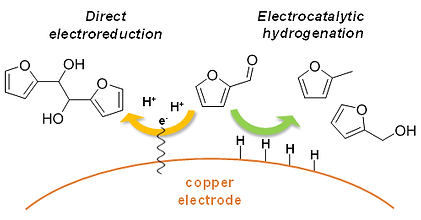
Biomass provides us with an abundant and renewable source of energy, chemicals and materials. Electrochemical reduction of biomass-derived platform chemicals is an emerging route for the sustainable production of fuels and chemicals, however, understanding gaps between reaction conditions, underlying mechanisms, and observed products have limited the rational design of active, stable, and selective catalyst systems.
We started our research on selective electrochemical reduction of levulinic acid (LA) to valeric acid (VA) or γ-valerolactone (gVL) on a non-precious Pb electrode in a polymer electrolyte membrane electrocatalytic flow cell reactor, which demonstrated very high yield of VA (>90%) and high faradaic efficiency (>86%). Electrode potential and electrolyte pH were found to tune the reduction products.
We explored the mechanisms of the electrochemical reduction of furfural, an important biobased platform molecule and model for aldehyde reduction, through a combination of voltammetry, preparative electrolysis, thiol-electrode modifications, and kinetic isotope studies. We found that the two isomers of hydrofuroin are generated from a direct electroreduction mechanism, while furfuryl alcohol and methyl furan are produced from electrocatalytic hydrogenation (ECH). Understanding the underlying mechanisms enabled us to manipulate the products of furfural reduction by rationally tuning the electrode potential, electrolyte pH, and furfural concentration to promote selective formation of important biobased polymer precursors and fuels. Our current research is focused on detailed mechanism study of C=O reduction, as well as development of electrochemical flow cells that can integrate the oxidation and reduction of biorenewable compounds for biopolymer synthesis. This research is collaborated with Prof. JP Tessonnier.
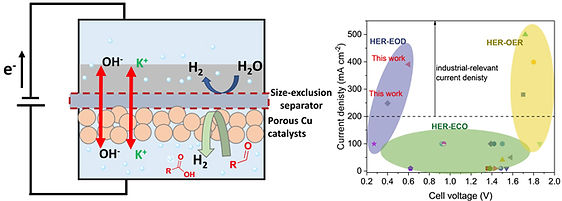
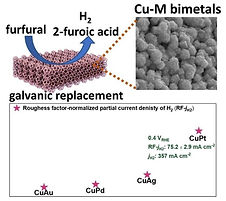
Recently we found electrochemical oxidation of furfural on Cu or Ag electrodes at low anodic potential can evolve H2, which enabled us to develop a membraneless, non-noble metal catalysts-based flow cell for bipolar H2 production from biorenewables aldehydes and water, and achieved industrial-level current densities at cell voltages much lower than conventional water electrolysis.
We are also working on the development of flow paired electrolyzers for efficient production of chemicals and monomers from distributed, inexpensive, renewable furanic feedstock. We are collaborating with Prof. Eric Cochran to develop new furanic polyesters.
Collaborating with our NSF-EFRI team (Profs. JP Tessonnier, Luke Roling, Eric Cochran, Sarah Ryan), we are rationally designing paired flow electrolyzers for electrocatalytic hydrogenation (ECH) of biomass-derived muconic acid (MA) to 3-hexenedioic acid (HDA) at the cathode, and halogen-mediated oxidation of MA or HDA at the anode.
Sponsors: NSF CBET 1512126, Iowa Energy Center (IEC) Opportunity Fund (2016), NSF CBET 1947435, NSF EFMA 2132200
Selected Publications:
Le Xin, Zhiyong Zhang, Ji Qi, David Chadderdon, Yang Qiu, Kayla Warsco, Wenzhen Li, Electricity storage in biofuels: Electrocatalytic conversion of levulinic acid to valeric acid and γ-valerolacetone, ChemSusChem, 2013, 6, 674-686. full article
Yang Qiu, Le Xin, David Chadderdon, Ji Qi, Changhai Liang, Wenzhen Li, Integrated electrocatalytic processing of levulinic acid and formic acid to biofuel intermediate valeric acid, Green Chemistry, 2014, 30, 7893-7901. full article
Xiaotong Chadderdon, David Chadderdon, John Matthiesen, Yang Qiu, Jack Carraher, Jean-Philippe Tessonnier, Wenzhen Li, Mechanisms of furfural reduction on metal electrodes: Distinguishing pathways for selective hydrogenation of bioderived oxygenates, Journal of the American Chemical Society, 2017, 139 (40), 14120-14128. DOI: 10.1021/jacs.7b06331
Xiaotong Chadderdon, David Chadderdon, Tony Pfennig, Brent Shanks and Wenzhen Li*, Paired Electrocatalytic Hydrogenation and Oxidation of 5-(Hydroxymethyl)furfural for Efficient Production of Biomass-derived Monomers, Green Chemistry, 2019, DOI: 10.1039/C9GC02264C.
Hengzhou Liu, Ting-Han Lee, Yifu Chen, Eric Cochran*, Wenzhen Li*, Paired electrolysis of 5-(hydroxymethyl)furfural in flow cells with high-performance oxide-derived silver cathode, Green Chemistry, 2021, DOI: 10.1039/D1GC00988E
Hengzhou Liu, Ting-Han Lee, Yifu Chen, Eric Cochran*, Wenzhen Li*, Paired and tandem electrochemical conversion of 5(hydroxymethyl)furfural using membrane-electrode-assembly-based electrocatalytic systems, ChemElectroChem, 2021, DOI: 10.1002/celc.202100662.
Hengzhou Liu, Deep Patel, Yifu Chen, Jungkuk Lee, Ting-Han Lee, Sarah D Cady, Eric Cochran, Luke Roling*, Wenzhen Li*, Unraveling Furfural Electro-Reductive Mechanisms via Tailoring Interfacial Environments, ACS Catalysis, 2022, 12, 14072-14085. 10.1021/acscatal.2c03163.
Hengzhou Liu#, Naveen Agrawal#, Arna Ganguly#, Yifu Chen, Jungkuk Lee, Jiaqi Yu, Wenyu Huang, Mark Mba-Wright*, Michael Janik*, Wenzhen Li*, Ultra-Low Voltage Bipolar Hydrogen Production from Biomass-Derived Aldehydes and Water in Membrane-Less Electrolyzers, Energy & Environmental Science, 2022, 15, 4175-4189. DOI: 10.1039/D2EE01427K.
Hengzhou Liu, Jiaqi Yu, Yifu Chen, Jungkuk Lee, Wenyu Huang, Wenzhen Li*, Cu-based Bimetallic Catalysts for Electrocatalytic Oxidative Dehydrogenation of Furfural with Practical Rates, ACS Applied Materials & Interfaces, 2023, 15, 37477-37485. doi/10.1021/acsami.3c06783
CO2 Capture and Electro-Reduction to Valuable Chemicals

The increase in atmospheric CO2 concentration from 280 to 420 ppm since the early 19th century has led to an average global temperature increase of ~1.2°C, leading to severe global warming and climate change issues. Worldwide energy-related CO2 emissions are currently at the level of 40 Gt. In order to keep within the 2°C goal set by the Paris agreement, approximately 10 Gt CO2 per year must be captured, with up to 160 Gt CO2 stored by 2050. Current efforts are focused on capturing and sequestering CO2 from point sources, e.g., flue gas with higher CO2 concentrations (10%), from over 1000 of the largest power plants that emit ~20% of total global fossil fuel CO2 emissions.
The electrocatalytic reduction of CO2 is a critical goal that could positively impact the global carbon balance by recycling CO2 into usable chemicals/fuels, thus attracting enormous research attention. Despite great breakthroughs in CO2 electro-reduction has been made in recent years, energy-efficient CO2 electro-reduction to selective production of targeted products (e.g. ethylene, formate, syngas) that can operate at low cell potentials (high energy efficiency) with high current density (high production rate) in a flow electrolysis mode remains a big challenge.
We are exploring using green ammonia to capture CO2 and then upgrading ammonium bicarbonate (NH4HCO3) to higher-valued chemicals. Our research goal is to develop earth-abundant metal catalysts for electro-reduction of captured CO2, optimize flow electrolyzer components and electrolytic conditions, and explore novel product separation methods, so as to design efficient paired electrolyzers for upgrading waste/residual carbons to valuable chemicals. We collaborate with Prof. Shuang Gu on NH3-assisted CO2 capture, and Prof. Mark Mba-Wright on TEA and LCA studies of the paired flow electrolyzers.
Compared to traditionally used CO2 capture reagent MEA (monoethanolamine), NH3 has a cheaper price as compared to MEA and base (e.g. KOH), and higher CO2-capturing capacity as it doubles the stoichiometric ratio of CO2 to the capturing agent. More importantly, release of CO2 from NH4HCO3 requires much lower energy than that from MEA-CO2 or KHCO3, as indicated by their decomposition temperatures: 36, 120, and 150 °C for NH4HCO3, MEA-CO2, and KHCO3, respectively. We found an ammonium bicarbonate (NH4HCO3)-fed electrolyzer outperforms the state-of-the-art KHCO3 electrolyzers largely because of its favorable thermal decomposition property, which allows for a 3-fold increase in the in situ CO2 concentration, a maximum 23% increase in formate faradaic efficiency, and a 35% reduction in cell voltage by substituting BPM with an anion exchange membrane (AEM). In addition, we have experimentally tested the remained NH4+ (aq) and lost NH3 (g) by NMR. At 100 mA/cm2, negligible NH3 loss was observed in the electrolyzer, since the amount of NH4+ (aq) was virtually unchanged in the NH4HCO3 solution at each hour interval and the escaped NH3 was merely 0.07% (in NH4+ equivalent) among the total NH4HCO3 detected in the NH3-absorbing solution.
We are working on electro-reduction of CO2 from CO2 capture liquids (focused on NH4HCO3) to other valuable chemicals (e.g. CO/H2, C2H4, etc), and are also designing innovative flow electrolyzers for pairing electrocatalytic reduction of CO2 at the cathode, and oxidation of locally distributed, renewable/residual carbons (e.g. glycerol, furfural) at the anode for continuous production of targeted valuable chemicals at low temperature, ambient pressure and mild pH conditions.
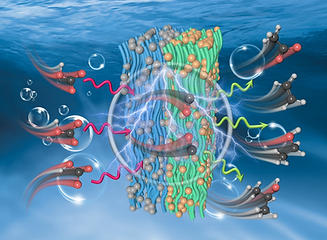
We are also studying electro-reduction of CO2 insitu generated from alkali capture bicarbonate solution (KHCO3). Electroreduction of bicarbonate to multicarbon products is achieved with applying Cu/Ag bilayered electrodes to increase local CO coverage, and pH and tailored microenvironment, as well as suppress the hydrogen evolution reaction. Bilayered Cu/Ag electrodes achieve a maximum C2+ faradaic efficiency of 41.6 % (the highest among all reported bicarbonate electroreduction studies) at the current density of 100 mA/cm2. Our current research is on investigating the effects catalyst/electrode structure, cation effects, pH and other conditions on electro-reduction of bicarbonate solution.
Sponsors: IEC Competitive Fund (2020), USDA-NIFA 2021-67021-34650, ISU VPR-PIRI (2022), NSF ECO-CBET 2219162
Selected Publication:
Jungkuk Lee, Hengzhou Liu, Yifu Chen, Wenzhen Li*, Bismuth Nanosheets Derived by In-Situ Morphology Transformation of Bismuth Oxides for Selective Electrochemical CO2 Reduction to Formate, ACS Applied Materials & Interfaces, 2022, 14, 14210-14217. DOI: 10.1021/acsami.1c25217
Jungkuk Lee, Hengzhou Liu, Wenzhen Li*, Tailoring Microenvironment in Bilayer Electrode with Copper and Silver Nanocatalysts for Bicarbonate Electroreduction to Multicarbon Products, ChemSusChem, Cover article, 2022, 10.1002/cssc.202201329.
Hengzhou Liu, Yifu Chen, Jungkuk Lee, Shuang Gu, Wenzhen Li*, Ammonia-Mediated CO2 Capture and Electroreduction to Formate, ACS Energy Letters, 2022, 7, 4483–4489. 10.1021/acsenergylett.2c02247
Electrochemical Synthesis of Ammonia and Nitrogen Fertilizers
Haber-Bosch ammonia synthesis is a mature industrial process, through which over 150 million tons of ammonia were produced per year for the fertilizer industry to support the large global population growth over the past century. However, this process consumes about 1.6% of all global energy output and emits ~1.5% of global CO2 (1.0% global GHG) emission. As an alternative, ammonia can be electrochemically synthesized directly from air and water without the need for harsh conditions (high temperature/pressure) and costly reactants such as high-purity hydrogen. Ammonia electrosynthesis has been proposed an innovative and transformative technology, which is fundamentally free of the problems of thermal synthesis, particularly considering its modular, flexible, and decentralized features. However, currently ammonia electrosynthesis has a very low Faradaic efficiency (electron efficiency, i.e. <5%), and production rate, (for example 10-9 molNH3/cm2-electrode/s). Great research breakthroughs in electrochemical materials and technologies may be used in electrosynthesis of ammonia.
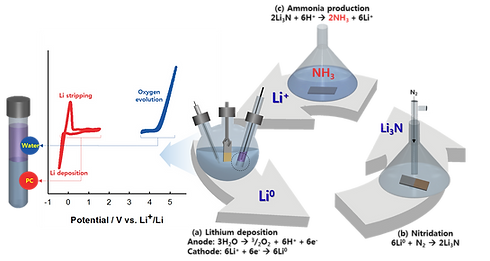
We have developed a membrane-free approach based on the immiscibility of aqueous/organic electrolytes for lithium electro-deposition, which can be utilized for subsequent nitridation and ammonia synthesis. We found that a biphasic system of aqueous 1 M LiClO4 and 1 M LiClO4/propylene carbonate reinforced with PMMA (poly(methyl methacrylate)) acts the same as a LISICON-based aqueous/organic hybrid electrolyte system, but without any physical membrane. We did 15N2 experiment to confirm ammonia was synthesized from N2 and demonstrated a fairly high Faradaic efficiency (FE) of 57.2% and a production rate of 1.21 × 10−9 mol/cm2/s for ammonia synthesis,
.jpg)
Our first research was to verify ammonia is truly synthesized from N2 gas, not other N-impurities. With our collaboration with Prof. Shuang Gu (from Wichita State University) and Prof. Stuart Licht (from George Washington University), we recently found substantial levels of nitrate-N and nitrides-N unexpectedly existed in some commercial metal oxides (for example, 563±21ppm-N in Alfa Aesar-45007) and metallic irons (for example, 7,297±99ppm-N in Alfa Aesar-40337), respectively. We offered a simple two-step procedure (alkaline treatment for NOx− or acidic treatment for nitrides, followed by either HPLC or UV–vis analysis) as a reliable protocol for screening NOx−/nitrides impurities in catalyst materials. Our work was published in Nature Catalysis as an Analysis article, and a folliwng perspective article was published in Trends in Chemistry.
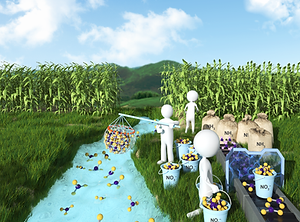

Sustainably recovering waste reactive nitrogen Nr (such as nitrate) and converting it into ammonia (NH3) could mitigate the environmental impacts of Nr, while reducing the NH3 demand from the carbon-intensive Haber–Bosch process. In this work, high-performance NO3− to NH3 conversion was achieved in a scalable, versatile, and cost-effective membrane-free alkaline electrolyzer (MFAEL): a remarkable NH3 partial current density of 4.22 ± 0.25 A /cm2 with a faradaic efficiency of 84.5 ± 4.9%. The unique configuration of MFAEL allows for the continuous production of pure NH3-based chemicals (NH3 solution and solid NH4HCO3) without the need for additional separation procedures. We have shown that the MFAEL system is capable of converting both inorganic and organic forms of nitrogen (e.g. nitrate, nitrite, urea, amino acids, proteins, algae, etc) to NH3 with ~100% conversion and selectivity, and are scaling up the MFAEL system. Our techno-economic analysis (TEA) revealed the economic competitiveness of upcycling waste N from dilute sources by combining nitrate reduction in MFAEL and a low-energy cost electrodialysis process for efficient nitrateconcentration.
Collaborating with our NSF EPSCoR II team (Profs. Michael Catellano, Liang Dong, Fallys Masambuka-Kanchewa, and Wichita State PIs), we are currently working on electrochemical synthesis of green fertilizers (focused on urea) from agricultural waste nitrogen (e.g. nitrate) and CO2, with the goals of promote N2O- and CO2-relieved nitrogen fertilizers (green urea as the focus) with economic resilience and environmental consciousness as an innovative way to mitigate the challenges posed upon climate change-threatened Midwest farming and ranching communities.
Sponsors: ARPAe-REFUEL (Subcontrats), IEC Cost-Share Funds (2017), ISU VPR-PIRI (2022), NSF EPSCoR 2316481
Selected publications:
Kwiyong Kim, Yifu Chen, Jong-In Han, Hyung Chul Yoon, and Wenzhen Li*, Lithium-mediated Ammonia Synthesis from Water and Nitrogen: A Membrane-free Approach Enabled by Immiscible Aqueous/Organic Hybrid Electrolyte System, Green Chemistry, 2019, 21, 3839–3845, DOI: 10.1039/C9GC01338E.
Yifu Chen, Hengzhou Liu, Nguon Ha, Stuart Licht*, Shuang Gu,* Wenzhen Li*, Revealing Nitrogen-Containing Species in Commercial Catalysts Used for Ammonia Electrosynthesis, Analysis article, Nature Catalysis, 2020, 2020, 10.1038/s41929-020-00527-4.
Usman Shahid#, Yifu Chen#, Shuang Gu*, Wenzhen Li*, Minhua Shao*, Electrochemical Nitrogen Reduction: An Intriguing but Challenging Quest, Trends in Chemistry, 2022, 4, 142-156.
https://doi.org/10.1016/j.trechm.2021.11.007
Yifu Chen, Pouya Ammari-Azar, Hengzhou Liu, Jungkuk Lee, Yu Xi, Michael Castellano, Shuang Gu*, Wenzhen Li*, Sustainable Waste-Nitrogen Upcycling Enabled by Low-Concentration Electrodialysis and High-Performance Ammonia Electrosynthesis, EES Catalysis, 2023, 1, 504-515. DOI: 10.1039/D3EY00058C.
Nitrogen Cycle Electrocatalysis
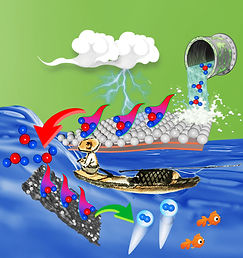
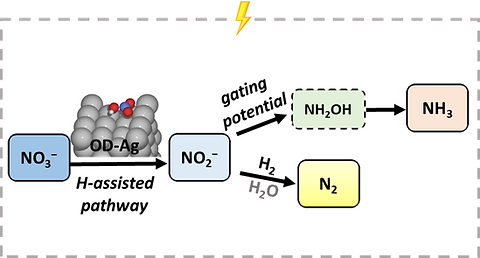
The heavy use of nitrogen fertilizer has introduced significant reactive nitrogen (in particular nitrate) in water bodies (> 53% lost in fertilizer practice), which led to severe water pollution, such as eutrophication, hypoxia, and harmful algal blooms in estuarine and near-shore areas. Compared with heterogeneous catalysis, the electrochemical denitrification (nitrate reduction) process has several unique advantages, including: direct removal of nitrate to clean N2 gas (closing the nitrogen cycle) or hydroxylamine (a higher-valued chemical), no need of H2, low energy costs, readily integration with renewable wind energy, flexible and modular electrochemical reactors with low capital costs.
We are studying the fundamental mechanisms of electrocatalytic reduction of nitrate (and nitrite) to dinitrogen by combining experimental and DFT computation approaches (with collaboration with Prof. Luke Roling), and based on new understanding, to engineer the composition and structure nitrate reduction catalysts to close N-cycle, one of the top identified engineering challenges.
We are interested in elucidating the similarities and differences between electrocatalytic reduction and heterogeneous catalytic reduction (by H2) of nitrate, and electrochemical upgrading of concentrated nitrate to higher-valued chemicals (e.g. hydroxylamine), and nitrogen fertilizers (e.g. urea) Our collaborators are Prof. Shuang Gu in selective concentration of nitrate and Prof. Mark Mba-Wright in TEA and LCA studies of electrochemical upgrading of nitrate from waste streams to valuable chemicals or fertilizers.
Sponsors: NSF CHE 2036944, Iowa Regents Innovation Fund (RIF, 2021), NSF EPSCoR 2316481
Selected publications:
Hengzhou Liu, Jaeryul Park, Yifu Chen, Yang Qiu, Yan Cheng, Kartik Srivastava, Shuang Gu, Brent H. Shanks, Luke T. Roling*, Wenzhen Li*, Electrocatalytic Nitrate Reduction on Oxide-Derived Silver with Tunable Selectivity to Nitrite and Ammonia, ACS Catalysis, 2021, 11, 8431-8442. DOI: acscatal.1c01525
Paired Electrolyzers To Addressing Critical Environmental and Sustainability Challenges
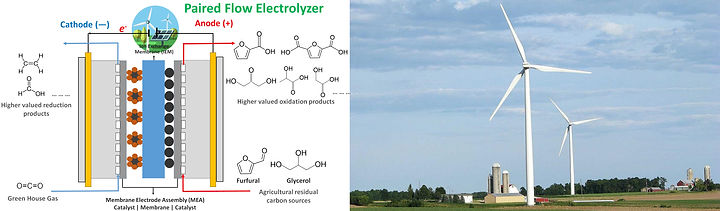
A paired electrolyzer separately produces two valuable chemicals by oxidizing and reducing feedstocks fed to the anode and cathode, thus it can make substantial gains in terms of economic feasibility and energy efficiency, including: 1) The thermodynamic potential of oxidation of an organic compound (e.g. –OH group in glycerol) is generally <0.2V, much lower than water oxidation (oxygen evolution reaction OER, 1.23V), normally coupled at the anode, increasing the operation voltage. O2 is less valuable. Replacing OER by organic oxidation represents 60%-80% saving in input energy. 2) The theoretical maximum electron efficiency (in terms of moles of products per unit charge moved in the cell) is theoretically 200%, instead of 100% for an unpaired electrolyzer. 3) The paired electrolyzers can lower capital and operation costs by reducing the number of reactors, extra processing and separation equipment required. It is worth mentioning that the chloro-alkali process, the largest electrochemical industry, indeed operates in paired electrolyzers for coproduction of chlorine at the anode, and H2 and alkali at the cathode. Furthermore, paired electrolyzers can accommodate two membranes (e.g. AEM, CEM) to combine chemical production and separation to achieve cost-effective, distributed chemical manufacturing by reducing product separation costs.
We started our research on pairing electro-oxidation and electro-reduction of biomass-derived compounds in flow electrolyzers. Recently we are moving to pair electro-oxidation / electro-reduction of small molecules/ions (e.g. H2, O2, H2O, CO2, CO, NO3-, NO2-, Cl-) with significant energy, environmental and sustainability meanings.
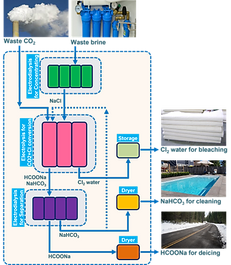
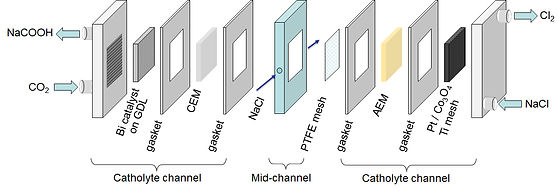
Chloride pollution has brought increasing concerns as it harms our environment, contaminates drinking water, and degrades transportation infrastructure. Chloride is toxic to aquatic life in freshwater ecosystems, especially for amphibians’ growth and reproduction (frogs, toads, salamanders). As an indispensable link to the healthy aquarium/terrarium ecological systems, amphibians play a critical role: they eat insect pests helping agriculture and control mosquitos benefiting human/animal health. In some area, chloride pollution has found its way to compromise drinking water supply, threatening public health. Chloride is also corrosive to roads, bridges, vehicles, and other transportation infrastructure, leading to an annual loss of $23.4 billion every year. On the other hand, the regeneration of water softeners discharges significant amount of waste brine waters containing high-concentration salt (typically, 55 mM NaCl and 34 mM Ca/MgCl2), contributing to the urban chloride pollution.
We hypothesize that 1) the proactive control of road/waste salt application/discharges can alleviate the growing chloride pollution in urban areas before catastrophic ecological/environmental failure; 2) the adequate replacement of road salt with the eco-friendly yet economic HCOONa can ease up the chloride pollution and its associated road damage and property loss; and 3) the combination of waste CO2 reduction and waste chloride oxidation can synergistically address chloride pollution issues with reduced CO2 emission in a paired electrolyzer.
Collaborating with our NSF ECO-CBET team (co-PI Peter Taylor, Wichita State PIs), we study pairing chlorine evolution reaction (ClER) and CO2 reduction reaction (CO2RR) in innovative flow electrolyzer: the overall reaction: 2CO2 + 2NaCl + H2O = HCOONa + NaHCO3 + Cl2. We are also interested in combining CO2 capture with CO2RR, which is paired with ClER. Rational design double-membrane flow cell is also a focus of this research project.
Sponsor: NSF CBET 1947435, NSF ECO-CBET 2219162
Selected publications:
Hengzhou Liu, Wenzhen Li*, Recent advances in paired electrolysis of biomass-derived compounds towards co-generation of value-added chemicals and fuels, Current Opinion in Electrochemistry, 2021, 30, 100795. https://doi.org/10.1016/j.coelec.2021.100795
Hengzhou Liu, Ting-Han Lee, Yifu Chen, Eric Cochran*, Wenzhen Li*, Paired electrolysis of 5-(hydroxymethyl)furfural in flow cells with high-performance oxide-derived silver cathode, Green Chemistry, 2021, 23, 5056-5063. DOI: 10.1039/D1GC00988E
Tianlei Li, Hengzhou Liu, Jiaqi Yu, Yifu Chen, Wenyu Huang, Wenzhen Li*, MOF-derived Co3O4/Nitrogen-doped carbon for efficient Chlorine-mediated Ethylene Oxidation, Green Chemistry, 2023, 25, 1982-1990. DOI: 10.1039/D2GC04508G
Basil Rawah, Mohammad Albloushi, Wenzhen Li*, Electro-Synthesis of Pure Aqueous H2O2 on Nitrogen-Doped Carbon in a Solid Electrolyte Flow Cell without Using Anion Exchange Membrane, Chemical Engineering Journal, 2023, 466, 143282. https://doi.org/10.1016/j.cej.2023.143282
HER / HOR Electrocatalysis
Hydrogen evolution reaction (HER) and hydrogen oxidation reaction (HOR) are primary reactions associated with aqueous electrochemistry. HER and HOR find important uses not only in H2/O2 fuel cells and water electrolyzers, but also potentially in ammonia production and CO2 conversion electrolyzers, in which HOR may be coupled at the anode in cases where H2 sources are abundantly available, while HER may affect CO2, nitrate, carbonyl group reduction performances. By switching from an acid electrolyte to alkaline electrolyte, the large overpotentials of oxygen reduction/evolution reaction (ORR/OER) - the long-time scientific challenge for fuel cells and electrolyzers - can be alleviated, however, the HER and HOR become sluggish in high pH electrolyte, thus more efficient HER/HOR electrocatalysts need to be explored.
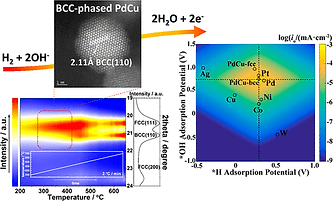
We have investigated HOR over bimetallic electrocatalysts for alkaline electrolyte-based fuel cells and ammonia electrolyzers, towards the goal of understanding the relationship between composition and structure of bimetallic catalysts and their electrocatalytic activity by combining experimental and theoretical DFT studies.
A long-term research goal is to acquire fundamental understanding the of similarities and differences between electrocatalytic hydrogenation (ECH) and heterogeneous catalytic reduction of small molecules, such as carbonyl group, CO2, NO3-, thus enabling advanced technologies for efficient energy conversion and distributed chemical manufacturing.
Selected publications:
Yang Qiu, Le Xin, Yawei Li, Ian T McCrum, Fangmin Guo, Tao Ma, Yang Ren, Qi Liu, Lin Zhou, Shuang Gu, Michael J Janik, Wenzhen Li*, BCC-Phased PdCu Alloy as a Highly Active Electrocatalyst for Hydrogen Oxidation in Alkaline Electrolytes, Journal of the American Chemical Society, 2018, 140 (48), 16580-16588. DOI:10.1021/jacs.8b08356
Other Research
We have been actively researching electrocatalysis, electrodes, and electrochemical devices for many years. Our past research projects include selective electrocatalytic oxidation of alcohols, direct alcohol fuel cells, direct carbohydrazide fuel cells, oxygen reduction and evolution reactions, photoelectrolysis of biorenewables, advanced catalytic materials, electrochemical processing of biorenewables, and they can be found in our published articles.
We gratefully acknowledge our current and previous sponsors:

I hope you enjoy surfing our website, thanks for your interest! Go Cyclones!
